使用者:PhiLiP/太陽系的形成與演化假說史

從已知最早的文獻開始,人類便一直在探索着世界的起源與結局;然而,在從古至今的絕大多數時間裡,人們都沒有試圖讓這樣的理論與「太陽系」的存在產生聯繫,只因為今日我們所理解的太陽系的存在,在當時幾乎沒有人知曉或相信。邁向太陽系的形成與演化理論的第一步,是對日心說的廣泛認同:這一模型將太陽放置在了整個系統的中心,而地球則在軌道上圍繞之作轉動。這一觀念曾醞釀有千年之久,但直到17世紀末才為公眾所廣泛接受。「太陽系」一詞有記錄以來最早的一次使用,出現在1704年。[1]
現今被接受的假說
[編輯]
星雲假說是至今以來最為人們所廣泛接受的行星形成理論。這一假說認為,太陽系形成於46億年前的一個橫跨數光年的巨大分子雲的引力坍縮。分子雲的坍縮造就了幾顆恆星,其中包括有太陽。構成太陽系的所有氣體的質量要略低於太陽本身。絕大多數的質量都被太陽系核心所吸收,並從而形成了太陽;剩餘的質量則形成了扁平的原行星盤,並最後形成了太陽系的各大行星與其他天體。
就像太陽與行星的誕生那樣,他們總有一天會走向死亡。隨着太陽年歲的增長,它將逐漸冷卻,並膨脹成為一顆直徑大過現在許多倍的紅巨星,其後,它將會拋棄其外殼(並形成行星狀星雲),成為恆星末期階段的白矮星。太陽系的行星將跟隨太陽演化的過程而變化;一部分將會被毀滅,其餘的則會被拋入星際空間;但是,經過足夠的時間後,圍繞太陽旋轉的天體最終都將不復存在。
形成假說
[編輯]1734年,Emanuel Swedenborg第一次提出了星雲假說[2],並由Immanuel Kant在1755年加以詳述與擴展。1796年,Pierre-Simon Laplace亦獨立地提出了相似的理論。[3]
1749年,Georges-Louis Leclerc, Comte de Buffon提出了行星形成的一種觀點:他認為,太陽曾與一顆彗星發生過碰撞,碰撞中散射出了形成行星的物質。然而,Laplace於1796年證偽了這一觀點,並得出結論認為,以該種方式形成的行星,最終都將會墜落到太陽表面。在今日,通常都認為,通過此種方式創造出太陽系所需的質量遠大於彗星的質量。[4]Laplace認為,行星近圓形的軌道是得出其形成原因所必需要考慮的因素。[4]
1755年,Immanuel Kant推測,所觀測到的星雲有可能實際上是恆星與行星形成的場所。1796年,Laplace詳細地描述到,星雲會坍塌成為恆星,隨後,剩下的物質會逐漸地向外部擴展形成一個扁平的盤,並最後形成行星。[4]
其他的理論
[編輯]星雲假說首要面臨的障礙是角動量的問題:如果太陽的確是通過星雲的坍塌而形成,那麼行星圍繞它轉動的速度將遠比現實中的慢。而實際上在整個系統中,儘管太陽有着幾乎99%的質量,但卻只占有1%的角動量。[5]
Attempts to resolve the angular momentum problem led to the temporary abandonment of the nebular hypothesis in favour of a return to "two-body" theories.[4] For several decades, many astronomers preferred the tidal or near-collision hypothesis put forward by James Jeans in 1917, in which the planets were considered to have been formed due to the approach of some other star to the Sun. This near-miss would have drawn large amounts of matter out of the Sun and the other star by their mutual tidal forces, which could have then condensed into planets.[4]</ref> However, in 1929 astronomer Harold Jeffreys countered that such a near-collision was massively unlikely.[4] Objections to the hypothesis were also raised by the American astronomer Henry Norris Russell, who showed that it ran into problems with angular momentum for the outer planets, with the planets struggling to avoid being reabsorbed by the Sun.[6]
In 1944, the Soviet astronomer Otto Schmidt proposed that the Sun, in its present form, passed through a dense interstellar cloud, emerging enveloped in a cloud of dust and gas, from which the planets eventually formed. This solved the angular momentum problem by assuming that the Sun's slow rotation was peculiar to it, and that the planets did not form at the same time as the Sun.[4] However, this hypothesis was severely dented by Victor Safronov who showed that the amount of time required to form the planets from such a diffuse envelope would far exceed the Solar System's determined age.[4]
In 1960, W. H. McCrea proposed the protoplanet theory, in which the Sun and planets individually coalesced from matter within the same cloud, with the smaller planets later captured by the Sun's larger gravity.[4] This theory has a number of issues, such as explaining the fact that the planets all orbit the Sun in the same direction, which would appear highly unlikely if they were each individually captured.[4]
The capture theory, proposed by M. M. Woolfson in 1964, posits that the Solar System formed from tidal interactions between the Sun and a low-density protostar. The Sun's gravity would have drawn material from the diffuse atmosphere of the protostar, which would then have collapsed to form the planets.[7] However, the capture theory predicts a different age for the Sun than for the planets,[來源請求] whereas the similar ages of the Sun and the rest of the Solar System indicate that they formed at roughly the same time.[8]
Reemergence of the nebular hypothesis
[編輯]
In 1978, astronomer A. J. R. Prentice revived the Laplacian nebular model in his Modern Laplacian Theory by suggesting that the angular momentum problem could be resolved by drag created by dust grains in the original disc which slowed down the rotation in the centre.[4][9] Prentice also suggested that the young Sun transferred some angular momentum to the protoplanetary disc and planetesimals through supersonic ejections understood to occur in T Tauri stars.[4][10] However, his contention that such formation would occur in toruses or rings has been questioned, as any such rings would disperse before collapsing into planets.[4]
The birth of the modern widely accepted theory of planetary formation—the Solar Nebular Disk Model (SNDM)—can be traced to the works of Soviet astronomer Victor Safronov.[11] His book Evolution of the protoplanetary cloud and formation of the Earth and the planets,[12] which was translated to English in 1972, had a long-lasting effect on the way scientists thought about the formation of the planets.[13] In this book almost all major problems of the planetary formation process were formulated and some of them solved. The Safronov's ideas were further developed in the works of George Wetherill, who discovered runaway accretion.[4] By the early 1980s, the nebular hypothesis in the form of SNDM had come back into favour, led by two major discoveries in astronomy. First, a number of apparently young stars, such as Beta Pictoris, were found to be surrounded by discs of cool dust, much as was predicted by the nebular hypothesis. Second, the Infrared Astronomical Satellite, launched in 1983, observed that many stars had an excess of infrared radiation that could be explained if they were orbited by discs of cooler material. Most astronomers today believe that the Sun's loss of angular momentum can be explained by loss of its matter to space in a phenomenon similar to the solar wind.[11]
Outstanding issues
[編輯]While the broad picture of the nebular hypothesis is widely accepted,[14] many of the details are not well understood and continue to be refined.
The refined nebular model was developed entirely on the basis of observations of our own Solar System because it was the only one known until the mid 1990s. It was not confidently assumed to be widely applicable to other planetary systems, although scientists were anxious to test the nebular model by finding of protoplanetary discs or even planets around other stars.[15] As of March 2008, the discovery of nearly 280 extrasolar planets[16] has turned up many surprises, and the nebular model must be revised to account for these discovered planetary systems, or new models considered.
Among the extrasolar planets discovered to date are planets the size of Jupiter or larger but possessing very short orbital periods of only a few days. Such planets would have to orbit very closely to their stars; so closely that their atmospheres would be gradually stripped away by solar radiation.[17][18] There is no consensus on how to explain these so-called hot Jupiters, but one leading idea is that of planetary migration, similar to the process which is thought to have moved Uranus and Neptune to their current, distant orbit. Possible processes that cause the migration include orbital friction while the protoplanetary disc is still full of hydrogen and helium gas[19] and exchange of angular momentum between giant planets and the particles in the protoplanetary disc.[20][21][22]
The detailed features of the planets are another problem. The solar nebula hypothesis predicts that all planets will form exactly in the ecliptic plane. Instead, the orbits of the classical planets have various (but small) inclinations with respect to the ecliptic. Furthermore, for the gas giants it is predicted that their rotations and moon systems will also not be inclined with respect to the ecliptic plane. However most gas giants have substantial axial tilts with respect to the ecliptic, with Uranus having a 98° tilt.[23] The Moon being relatively large with respect to the Earth and other moons which are in irregular orbits with respect to their planet is yet another issue. It is now believed these observations are explained by events which happened after the initial formation of the Solar System.[24] For instance, the large axial tilts of many planets may be explained by giant impacts with massive planetesimals early in the Solar System's evolution.[25]
Solar evolution hypotheses
[編輯]Attempts to isolate the physical source of the Sun's energy, and thus determine when and how it might ultimately run out, began in the 19th century. At that time, the prevailing scientific view on the source of the Sun's heat was that it was generated by gravitational contraction. In the 1840s, astronomers J. R. Mayer and J. J. Waterson first proposed that the Sun's massive weight causes it to collapse in on itself, generating heat, an idea expounded upon in 1854 by both Hermann von Helmholtz and Lord Kelvin, who further elaborated on the idea by suggesting that heat may also be produced by the impact of meteors onto the Sun's surface.[26] However, the Sun only has enough gravitational potential energy to power its luminosity by this mechanism for about 30 million years—far less than the age of the Earth. (This collapse time is known as the Kelvin-Helmholtz timescale.)[27]
Albert Einstein's development of the theory of relativity in 1905 led to the understanding that nuclear reactions could create new elements from smaller precursors, with the loss of energy. In his treatise Stars and Atoms, Arthur Eddington suggested that pressures and temperatures within stars were great enough for hydrogen nucei to fuse into helium; a process which could produce the massive amounts of energy required to power the Sun.[26] In 1935, Eddington went further and suggested that other elements might also form within stars.[28] Spectral evidence collected after 1945 showed that the distribution of the commonest chemical elements, carbon, hydrogen, oxygen, nitrogen, neon, iron etc, was fairly uniform across the galaxy. This suggested that these elements had a common origin.[28] A number of anomalies in the proportions hinted at an underlying mechanism for creation. Lead is heavier than gold, but far more common. Hydrogen and helium (elements 1 and 2) are virtually ubiquitous yet lithium and beryllium (elements 3 and 4) are extremely rare.[28]
Red giants
[編輯]While the unusual spectra of red giant stars had been known since the 19th century,[29] it was George Gamow who, in the 1940s, first understood that they were stars of roughly solar mass that had run out of hydrogen in their cores and had resorted to burning the hydrogen in their outer shells.[來源請求] This allowed Martin Schwarzschild to drew the connection between red giants and the finite lifespans of stars. It is now understood that red giants are stars in the last stages of their life cycles.
Fred Hoyle noted that, even while the distribution of elements was fairly uniform, different stars had varying amounts of each element. To Hoyle, this indicated that they must have originated within the stars themselves. The abundance of elements peaked around the atomic number for iron, an element that could only have been formed under intense pressures and temperatures. Hoyle concluded that iron must have formed within giant stars.[28] From this, in 1945 and 1946, Hoyle constructed the final stages of a star's life cycle. As the star dies, it collapses under its own weight, leading to a stratified chain of fusion reactions: carbon-12 fuses with helium to form oxygen-16; oxygen-16 fuses with helium to produce neon-20, and so on up to iron.[30] There was, however, no known method by which carbon-12 could be produced. Isotopes of beryllium produced via fusion were too unstable to form carbon, and for three helium atoms to form carbon-12 was so unlikely as to have been impossible over the age of the universe. However, in 1952 the physicist Ed Salpeter showed that a short enough time existed between the formation and the decay of the beryllium isotope that another helium had a small chance to form carbon, but only if their combined mass/energy amounts were equal to that of carbon-12. Hoyle, employing the anthropic principle, showed that it must be so, since he himself was made of carbon, and he existed. When the matter/energy level of carbon-12 was finally determined, it was found to be within a few percent of Hoyle's prediction.[31]
White dwarfs
[編輯]The first white dwarf discovered was in the triple star system of 40 Eridani, which contains the relatively bright main sequence star 40 Eridani A, orbited at a distance by the closer binary system of the white dwarf 40 Eridani B and the main sequence red dwarf 40 Eridani C. The pair 40 Eridani B/C was discovered by Friedrich Wilhelm Herschel on January 31, 1783;[32], p. 73 it was again observed by Friedrich Georg Wilhelm Struve in 1825 and by Otto Wilhelm von Struve in 1851.[33][34] In 1910, it was discovered by Henry Norris Russell, Edward Charles Pickering and Williamina Fleming that despite being a dim star, 40 Eridani B was of spectral type A, or white.[35]
White dwarfs were found to be extremely dense soon after their discovery. If a star is in a binary system, as is the case for Sirius B and 40 Eridani B, it is possible to estimate its mass from observations of the binary orbit. This was done for Sirius B by 1910,[36] yielding a mass estimate of 0.94 solar mass. (A more modern estimate is 1.00 solar mass.)[37] Since hotter bodies radiate more than colder ones, a star's surface brightness can be estimated from its effective surface temperature, and hence from its spectrum. If the star's distance is known, its overall luminosity can also be estimated. Comparison of the two figures yields the star's radius. Reasoning of this sort led to the realization, puzzling to astronomers at the time, that Sirius B and 40 Eridani B must be very dense. For example, when Ernst Öpik estimated the density of a number of visual binary stars in 1916, he found that 40 Eridani B had a density of over 25,000 times the Sun's, which was so high that he called it "impossible".[38]
Such densities are possible because white dwarf material is not composed of atoms bound by chemical bonds, but rather consists of a plasma of unbound nuclei and electrons. There is therefore no obstacle to placing nuclei closer to each other than electron orbitals—the regions occupied by electrons bound to an atom—would normally allow.[39] Eddington, however, wondered what would happen when this plasma cooled and the energy which kept the atoms ionized was no longer present.[40] This paradox was resolved by R. H. Fowler in 1926 by an application of the newly devised quantum mechanics. Since electrons obey the Pauli exclusion principle, no two electrons can occupy the same state, and they must obey Fermi-Dirac statistics, also introduced in 1926 to determine the statistical distribution of particles which satisfy the Pauli exclusion principle.[41] At zero temperature, therefore, electrons could not all occupy the lowest-energy, or ground, state; some of them had to occupy higher-energy states, forming a band of lowest-available energy states, the Fermi sea. This state of the electrons, called degenerate, meant that a white dwarf could cool to zero temperature and still possess high energy.
Planetary nebulae
[編輯]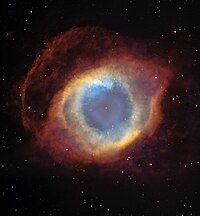
Planetary nebulae are generally faint objects, and none are visible to the naked eye. The first planetary nebula discovered was the Dumbbell Nebula in the constellation of Vulpecula, observed by Charles Messier in 1764 and listed as M27 in his catalogue of nebulous objects. To early observers with low-resolution telescopes, M27 and subsequently discovered planetary nebulae somewhat resembled the gas giants, and William Herschel, discoverer of Uranus, eventually coined the term 'planetary nebula' for them, although, as we now know, they are very different from planets.
The central stars of planetary nebulae are very hot. Their luminosity, though, is very low, implying that they must be very small. Only once a star has exhausted all its nuclear fuel can it collapse to such a small size, and so planetary nebulae came to be understood as a final stage of stellar evolution. Spectroscopic observations show that all planetary nebulae are expanding, and so the idea arose that planetary nebulae were caused by a star's outer layers being thrown into space at the end of its life.
Lunar origins hypotheses
[編輯]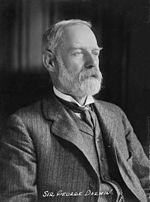
Over the centuries, many scientific hypotheses have been advanced concerning the origin of Earth's Moon. One of the earliest was the so-called binary accretion model, which concluded that the Moon accreted from material in orbit around the Earth left over from its formation. Another, the fission model, was developed by George Darwin, (son of Charles Darwin) who noted that, as the Moon is gradually receding from the Earth at a rate of about 4 cm per year, so at one point in the distant past it must have been part of the Earth, but was flung outward by the momentum of Earth's then–much faster rotation. This hypothesis is also supported by the fact that the Moon's density, while less than Earth's, is about equal to that of Earth's rocky mantle, suggesting that, unlike the Earth, it lacks a dense iron core. A third hypothesis, known as the capture model, suggested that the Moon was an independently orbiting body that had been snared into orbit by Earth's gravity.[42]
Apollo missions
[編輯]However, these hypotheses were all refuted by the Apollo lunar missions, which introduced a stream of new scientific evidence; specifically concerning the Moon's composition, its age, and its history. These lines of evidence contradict many predictions made by these earlier models.[42] The rocks brought back from the Moon showed a marked decrease in water relative to rocks elsewhere in the Solar System, and also evidence of an ocean of magma early in its history, indicating that its formation must have produced a great deal of energy. Also, oxygen isotopes in lunar rocks showed a marked similarity to those on Earth, suggesting that they formed at a similar location in the solar nebula. The capture model fails to explain the similarity in these isotopes (if the Moon had originated in another part of the Solar System, those isotopes would have been different), while the co-accretion model cannot adequately explain the loss of water (if the Moon formed in a similar fashion to the Earth, the amount of water trapped in its mineral structure would also be roughly similar). Conversely, the fission model, while it can account for the similarity in chemical composition and the lack of iron in the Moon, cannot adequately explain its high orbital inclination and, in particular, the large amount of angular momentum in the Earth/Moon system, more than any other planet–satellite pair in the Solar System.[42]
Giant impact hypothesis
[編輯]For many years after Apollo, the binary accretion model was settled on as the best hypothesis for explaining the Moon's origins, even though it was known to be flawed. Then, at a conference in Kona, Hawaii in 1984, a compromise model was composed that accounted for all of the observed discrepencies. Originally formulated by two independent research groups in 1976, the giant impact model supposed that a massive planetary object, the size of Mars, had collided with Earth early in its history. The impact would have melted Earth's crust, and the other planet's heavy core would have sunk inward and merged with Earth's. The superheated vapour produced by the impact would have risen into orbit around the planet, coalescing into the Moon. This explained the lack of water (the vapour cloud was too hot for water to condense), the similarity in composition (since the Moon had formed from part of the Earth), the lower density (since the Earth had formed from the Earth's crust and mantle, rather than its core, and the Moon's unusual orbit (since an oblique strike would have imparted a massive amount of angular momentum to the Earth/Moon system.[42]
Outstanding issues
[編輯]However, the giant impact model has been criticised for being too explanatory; it can be expanded to explain any future discoveries and as such, makes no predictions. Also, many claim that much of the material from the impactor would have ended up in the Moon, meaning that the isotope levels would be different, but they are not. Also, while water and many other volatile compounds are absent from the Moon's crust, many others, such as manganese, are not.[42]
Other natural satellites
[編輯]While the co-accretion and capture models are not currently accepted as valid explanations for the existence of the Moon, they have been employed to explain the formation of other natural satellites in the Solar System. Jupiter's Galilean satellites are believed to have formed via co-accretion,[43] while the Solar System's irregular satellites, such as Triton, are all believed to have been captured.[44]
參考文獻
[編輯]- ^ Solar [太陽]. etymoline. [2008-04-15] (英語).
- ^ Swedenborg, Emanuel. Principia, Volume 1. Opera Philosophica et Mineralia [哲學與礦物學作品]. 1734年.
- ^ See, T. J. J. The Past History of the Earth as Inferred from the Mode of Formation of the Solar System. Proceedings of the American Philosophical Society. 1909, 48: 119頁 [2006-07-23].
- ^ 4.00 4.01 4.02 4.03 4.04 4.05 4.06 4.07 4.08 4.09 4.10 4.11 4.12 4.13 M. M. Woolfson. The Solar System: Its Origin and Evolution. Physics Department, University of New York. 1993年, 34: 1-20頁 [2008-04-16].
|journal=
和|work=
只需其一 (幫助) - ^ Woolfson, M. M. Rotation in the Solar System. Philosophical Transactions of the Royal Society of London. 1984年, 313: 5頁.
- ^ Benjamin Crowell. 5. Conservation Laws. lightandmatter.com. 1998-2006.
- ^ J. R. Dormand & M. M. Woolfson. The capture theory and planetary condensation. Monthly Notices of the Royal Astronomical Society. 1971, 151: 307 [2008-04-16].
- ^ Weidenschilling, S. J.; Spaute, D.; Davis, D. R.; Marzari, F.; Ohtsuki, K. Accretional Evolution of a Planetesimal Swarm. Icarus. 1997, 128: 429–455 [2008-03-13]. doi:10.1006/icar.1997.5747.
- ^ Prentice, A. J. R. Origin of the solar system. I - Gravitational contraction of the turbulent protosun and the shedding of a concentric system of gaseous Laplacian rings. Moon and Planets. 1978, 19: 341–398.
- ^ Ferreira, J.; Dougados, C.; Cabrit, S. Which jet launching mechanism(s) in T Tauri stars?. Astronomy & Astrophysics. 2006, 453: 785. doi:10.1051/0004-6361:20054231. .
- ^ 11.0 11.1 Nigel Henbest. Birth of the planets: The Earth and its fellow planets may be survivors from a time when planets ricocheted around the Sun like ball bearings on a pinball table. New Scientist. 1991 [2008-04-18].
- ^ Safronov, Viktor Sergeevich. Evolution of the Protoplanetary Cloud and Formation of the Earth and the Planets. Israel Program for
Scientific Translations. 1972. ISBN 0706512251. 參數
|publisher=
值左起第19位存在換行符 (幫助) - ^ George W. Wetherill. Leonard Medal Citation for Victor Sergeevich Safronov. Meteoritics. 1989, 24: 347.
- ^ e. g.
- Kokubo, Eiichiro; Ida, Shigeru. Formation of Protoplanet Systems and Diversity of Planetary Systems. Astrophysical Journal. 2002, 581: 666. doi:10.1086/344105.
- Lissauer, J. J. Planet Formation, Protoplanetary Disks and Debris Disks. L. Armus and W. T. Reach, eds. (編). The Spitzer Space Telescope: New Views of the Cosmos 357. Astronomical Society of the Pacific Conference Series: 31. 2006.
- Zeilik, Michael A.; Gregory, Stephan A. Introductory Astronomy & Astrophysics 4th. Saunders College Publishing. 1998. ISBN 0030062284.
- ^ Planet Quest, Terrestrial Planet Finder. NASA Jet Propulsion Laboratory. [2008-02-01].
- ^ Jean Schneider. The extrasolar planets encyclopedia. Paris University. [2008-03-13].
- ^ Weaver, D.; Villard, R. Hubble Probes Layer-cake Structure of Alien World's Atmosphere. University of Arizona, Lunar and Planetary Laboratory (Press Release). 2007-01-31 [2007-08-15].
- ^ Ballester, Gilda E.; Sing, David K.; Herbert, Floyd. The signature of hot hydrogen in the atmosphere of the extrasolar planet HD 209458b. Nature. 2007, 445: 511. doi:10.1038/nature05525.
- ^ Benjamin Crowell. Vibrations and Waves. 2008 [2008-02-01].
- ^ Tsiganis, K.; Gomes, R.; Morbidelli, A.; Levison, H. F. Origin of the orbital architecture of the giant planets of the Solar System. Nature. 2005, 435 (7041): 459. doi:10.1038/nature03539.
- ^ Lissauer, J. J., Planet Formation, Protoplanetary Disks and Debris Disks, L. Armus and W. T. Reach (編), The Spitzer Space Telescope: New Views of the Cosmos 357, Astronomical Society of the Pacific Conference Series: 31, 2006
- ^ ">Fogg, M. J.; Nelson, R. P. On the formation of terrestrial planets in hot-Jupiter systems. Astronomy & Astrophysics. 2007, 461: 1195. doi:10.1051/0004-6361:20066171. .
- ^ Heidi B. Hammel. Uranus nears Equinox (PDF). Pasadena Workshop. 2006 [2008-03-13].
- ^ Frank Crary. The Origin of the Solar System. Colorado University, Boulder. 1998 [2008-03-13].
- ^ Adrián Brunini. Origin of the obliquities of the giant planets in mutual interactions in the early Solar System. Nature. 2006, 440 (7088): 1163–1165. doi:10.1038/nature04577. 已忽略未知參數
|month=
(建議使用|date=
) (幫助) - ^ 26.0 26.1 David Whitehouse. The Sun: A Biography. John Wiley and Sons. 2005.
- ^ Carl J. Hansen, Steven D. Kawaler, and Virgina Trimble. Stellar interiors: physical principles, structure, and evolution. New York: Springer. 2004: p. 4. ISBN 0-387-20089-4.
- ^ 28.0 28.1 28.2 28.3 Simon Mitton. Origin of the Chemical Elements. Fred Hoyle: A Life in Science. Aurum. 2005: 197–222.
- ^ Oscar Straniero, Roberto Gallino, and Sergio Cristallo. s process in low-mass asymptotic giant branch stars. Nuclear Physics A. 17 October 2006, 777 [2008-04-02]. doi:10.1016/j.nuclphysa.2005.01.011. 已忽略未知參數
|Pages=
(建議使用|pages=
) (幫助) - ^ J. Faulkner. Fred Hoyle, Red Giants and beyond. Astrophysics and Space Science. 2003, 285 (2): 339–339. doi:10.1023/A:1025432324828. 已忽略未知參數
|accessate=
(建議使用|access-date=
) (幫助) - ^ The Nuclear Physics Group. Life, Bent Chains, and the Anthropic Principle. The University of Birmingham. [2008-04-21].
- ^ Catalogue of Double Stars, William Herschel, Philosophical Transactions of the Royal Society of London 75 (1785), pp. 40–126
- ^ The orbit and the masses of 40 Eridani BC, W. H. van den Bos, Bulletin of the Astronomical Institutes of the Netherlands 3, #98 (July 8, 1926), pp. 128–132.
- ^ Astrometric study of four visual binaries, W. D. Heintz, Astronomical Journal 79, #7 (July 1974), pp. 819–825.
- ^ How Degenerate Stars Came to be Known as White Dwarfs, J. B. Holberg, Bulletin of the American Astronomical Society 37 (December 2005), p. 1503.
- ^ Preliminary General Catalogue, L. Boss, Washington, D.C.: Carnegie Institution, 1910.
- ^ The Age and Progenitor Mass of Sirius B, James Liebert, Patrick A. Young, David Arnett, J. B. Holberg, and Kurtis A. Williams, The Astrophysical Journal 630, #1 (September 2005), pp. L69–L72.
- ^ The Densities of Visual Binary Stars, E. Öpik, The Astrophysical Journal 44 (December 1916), pp. 292–302.
- ^ On the relation between the masses and luminosities of the stars, A. S. Eddington, Monthly Notices of the Royal Astronomical Society 84 (March 1924), pp. 308–332.
- ^ On Dense Matter, R. H. Fowler, Monthly Notices of the Royal Astronomical Society 87 (1926), pp. 114–122.
- ^ The Development of the Quantum Mechanical Electron Theory of Metals: 1900-28, Lillian H. Hoddeson and G. Baym, Proceedings of the Royal Society of London, Series A, Mathematical and Physical Sciences 371, #1744 (June 10, 1980), pp. 8–23.
- ^ 42.0 42.1 42.2 42.3 42.4 Paul D. Spudis. Whence the Moon?. The Once and Future Moon. Smithsonian Institution Press. 1996: 157–169.
- ^ Robin M. Canup and William R. Ward. Formation of the Galilean Satellites: Conditions of Accretion 124: 3404–3423. 2002 [2008-04-22]. doi:10.1086/344684. 已忽略文本「journalThe Astronomical Journal」 (幫助)
- ^ David Nesvorný, David Vokrouhlický and Alessandro Morbidelli. Capture of Irregular Satellites during Planetary Encounters. The Astronomical Journal. 2007, 133: 1962–1976 [2008-04-22]. doi:10.1086/512850.
Category:恆星天文學 Category:行星科學 Category:太陽系 Category:太陽系的動態理論 Category:天文學史 Category:物理學史